Introduction to Walk Like a Sloth: lessons in ground sloth locomotion
Getting Oriented
Like you, and unlike tree sloths and most other ground sloths, Megalonyx had five (5) toes on each foot. Your toes, like your fingers, have three (3) phalanges (segments) each, except your big toe and thumb which each have just two (2) phalanges. Megalonyx is the same, only the middle toe is the big toe, and has two (2) bones. In Megalonyx the proximal phalanx (the bone closer to the body) and medial phalanx (middle bone) of the 3rd toe are fused, so completely that all traces that they started out as two separate bones have vanished. What looks like the proximal phalanx is actually the third metatarsal.
Like yours, each of the sloth’s toes connects to the tarsals (“ankle” bones) via a metatarsal, but space gets cramped close to the ankle so the proximal ends of the metatarsals are beveled so they can pack together and glide across each other where they meet. The proximal end of the third metatarsal forms a “T” or “Y” with its cup (see photo at left); the cross bar is “up,” the top or dorsal side. A facet on each side, near the proximal dorsal surface, shows where this bone articulates with digits #2 and #4, demonstrating that this is a metatarsal and not a phalanx. (Phalanges only articulate fore and aft, never starboard or port.) The facets face slightly downward, and together the metatarsals form a shock-absorbing arch with #3 serving as the keystone. The distal (far) end of the metatarsal forms a vertical keel with two raised buttresses separated from the keel by a deep groove for articulating with the proximal end of the phalanx. The two bones fit together tightly, like pieces of a jigsaw puzzle. Try it. (If the joint isn’t tight, turn the phalanx over and try it in a different direction until it fits.) Add the ungual digit (claw) from lesson 4 to complete the toe http://slotsonlinecanada.ca/.
The claw can rotate about 55° on the fused phalanx. The proximal end of the claw forms a circular socket of over 180°, making a tight fit with the phalanx in any position. Bony projections on both the proximal dorsal and ventral ends of the claw hit a bony stop on the medial phalanx preventing the claw from over-extending or flexing. Compare this tight-fitting joint to that between the phalanx and metatarsal, called the metatarsophalangeal joint (MPJ). The distal end of the metatarsal forms a wider circle and the joint encompasses about 130°, with no bony stops. Jiggle the bone in the socket as you bend it. It’s very loose in comparison, especially extended or flexed, owing to the flatter curve of the metatarsal; it’s not a good fit. As in the sloth, all your phalanges can do is bend back and forth. Most of the flexibility you have in your digits to move sideways comes from the MPJ’s. However, flexible joints bring with them the danger of dislocating or straining the muscles and ligaments that keep them stable. That’s the risk for Megalonyx too, which is why this joint is abundantly reinforced with extensive ligaments, multiple layers of muscles and two amazing bones called sesamoids (not included in the set) which act as joint stabilizers and also boost the power of the digit.
Examine the proximal end of the fused phalanx and notice that the buttresses bordering the vertical socket are truncated (“chopped off,” see arrow) on their ventral ends. They don’t come to a point like they do on their dorsal ends. The flattened medial end is larger than the lateral end. Now examine the lower (ventral) end of the keel on the distal end of the metatarsal. There’s a slight indentation on both sides, larger again on the medial side. These landmarks show the resting position of two sesamoid bones that are a key part of the third metatarsophalangeal joint. Each is embedded in a tendon running from a muscle attached to the heel to the front claw and wrapped in a network of strong and flexible ligaments connecting them to the head of the metatarsal, the adjacent metatarsals, and each other. Together they create a sort of floating buttress for the joint which stabilizes it in any direction the claw moves–up, down or sideways, “filling in” the gap. The sesamoids also raise the ball of the foot, help absorb shocks and protect the metatarsal head and tendon. Finally, they act like pulleys to increase the mechanical advantage of the muscle. More about sesamoids below in Look closer.
Key points
#1 Before you identify the bones for students and bring in the claw, have them try to guess what they are. Most people new to bones mistake these toe bones for back bones (i.e. vertebrae). Show them how the joints work. Does your back just bend back and forth? Would a sloth’s? [No, they twist sideways too.] These bones can’t do that. New learners also forget that vertebrae have a tunnel running through them to protect the spinal cord. No tunnel in the toe bones! The bones aren’t nearly big enough either to be the vertebrae of an animal weighing over a ton. (see the vertebrae lesson) Don’t feel bad, nearly everyone makes this mistake.
#2 The bones of the third toe weren’t fused in every Megalonyx. It’s a characteristic seen more often in later individuals of the species than earlier ones. Fused or not though, Megalonyx needed the rigid support for this important claw (the biggest one). Even when the bones were separate, as they were in the animal pictured to the right, dorsal and ventral ridges on the medial phalanx (see arrows) extended over the articular surface of the proximal phalanx, preventing any flexing of the joint. Fused or unfused, these bones worked as a unit in the middle toe, tied together by the sesamoid network. (David et al., 1989)
#3 In Megalonyx the third toe takes the place of our big toe as the primary weight-bearing, balancing and locomotion complex. Your big toe has to support about 40% of your weight every time you push off of it; more when you run. (Finch, 2011) The stress on the sloth’s middle toe was probably similar. Fusing and shortening the phallanges gives the digit greater strength, more rigidity and provides better support. (Stock, 1917) Fusion of different foot bones was common in other lineages of ground sloths to strengthen the feet (McDonald, 1977), but rigidity in this particular digit was critical in Megalonyx because, like your big toe, it doesn’t merely support the animal. With its sesamoids it actually catapults the foot forward. (David et al., 1989)
Additional information
Foot bones of mammals generally fit together tightly like pieces of a puzzle. Dinosaurs had feet with many fewer bones than mammals and they were not nearly as well packed. Mammal feet act as a unit, with bones pressing against bones over wide areas of articulation; more bones and more stable bone interaction generally permit more complex movements with relatively more strength and agility. (Jensen, 1988)
Sesamoids are bones that form inside tendons where they pass over a joint. They help protect the tendon and prevent it from flattening when the joint bends, decreasing its mechanical advantage. Your kneecap is the best known example. By holding the tendons that extend your lower leg away from the center of the joint, knee caps increase the moment arm (improve “leverage”) by changing the direction of the pulling muscle.
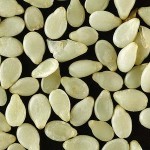
Sesame seeds (image courtesy of Wikipedia)
Sesamoids were named by the Roman physician and philosopher Galen, who was reminded of sesame seeds by their size and shape (in humans). Most of us have just 8 phalange sesamoids (two in each of our thumbs and big toes), but they can grow in any joint in response to injury, stress or genes. Bizarro (1921) found people with as many as seven (7), spread among the fingers of one hand, and five (5) among their toes. Individuals with as many as 42 sesamoids have been reported. (Sarin et al., 1999)
We are taught that humans have 206 bones as adults. That count includes the biggest sesamoid, the patella (knee cap), but none of the others. How come? Probably because the smaller sesamoids are easily lost or overlooked when bones are being recovered, plus their function was long dismissed as unimportant.
Look closer
The sesamoids of the sloth’s middle toe occupy the same position as they do in your big toe and play a similar role. In life your sesamoids are embedded in a tendon of the flexor halucis brevis muscle, literally “the short big-toe flexor,” (flexor digitorum brevis in sloths) which is anchored on the calcaneum (heel bone) and inserts on the distal phalanx.
The short flexor gets assistance from the flexor hallucis longus muscle, the “long big-toe flexor,” (flexor digitorum longus in sloths) which originates on the lower leg and forms a long tendon which runs under and around the calcaneum (heel bone) and down the length of the foot to insert at the base of the distal phalanx. You might suppose pressing your weight down on the toe with every step would pinch the tendon and prevent further contraction but it slides freely in the gap between the sesamoids.
The sesamoids are tied to each other with an intersesamoidal ligament. Transverse ligaments attach the sesamoids to the adjacent metatarsals. There’s also a connection to the dorsal extensor tendon. David et al. (1989) refer to the whole complex apparatus as the “sesamoid muscles,” likening their function to a catapult assisting in propulsion while at the same time serving to distribute and coordinate the forces needed in the forefoot for balance and propulsion.
Things to do
Toe-off like a sloth Talk a short stroll around the room. Can you feel yourself pushing off your big toe with every step forward? Toes are one of the mechanisms bipedal animals have to both smooth their walk and save energy. They work like shock absorbers, with your Achille’s tendon, to put a literal spring in your step. The tendon stretches as your foot hits the ground and springs back to help you push off your toes—doing about 15% of the work and saving energy, especially when toe-pushing is important, as in running. Savings are 50% in some animals! (Hildebrand, 1985) If you imagined Megalonyx upright at all, it was probably rocking back and forth like Godzilla in a cheap Japanese movie. Change that picture and put him on his toes!
Catapult like a sloth Like you, as the sloth stepped forward and planted his foot, his sesamoids were pressed into the ground, locking them in place. He didn’t lose his balance or control of the claw because he still had the tendon of the long muscle of the digit passing freely between the sesamoids and pressing the toe into the ground. As he continued forward the tendon between the sesamoids and heel stretched tight. When he shifted his weight and stepped forward on the opposite foot, it took the pressure off the sesamoids, and all the energy stored in the stretched tendon would flex the claw and fling the toe and foot backward, pushing the torso forward. Take another stroll around the room and try out your catapults. Remember, “Step, stretch, fling. Step, stretch, fling. Step, stretch, fling.”
Glide like a model Toes work with your knees to help you maintain a relatively constant height and reduce wasted effort bobbing up and down. That leaves more energy for propelling your body forward rather than lifting it needlessly. A little bouncing is unavoidable, but vertical displacement is normally limited to less than two inches when humans walk. (Inman, 1966)
Walk like a pirate You can’t remove your toes but you can mimic the effect of walking without them by locking your knee and ankle on one leg and walking on your heel. Without shock-absorbing toes your motion becomes jerky like a pirate with a peg leg. Notice how much more you go up and down with every step. No toe-springs saving you energy now!
Walk like an Egyptian The oldest known prosthetic device is a wood and leather big toe that was found on a female mummy identified as Tabaketenmut, discovered near Thebes and dating back to around 700-800 BC. (Finch, 2011) Scientists believe Tabaketenmut actually wore the device and it wasn’t just strapped on for her funeral. (ibid.) Many people believe you can’t walk if you lose your big toe but that’s not true. Lots of people lose their toes in accidents or due to diabetes (as did Tabaketenmut apparently) but they learn to walk again, albeit not as smoothly or efficiently. Big toes are important–try walking by keeping your weight on your other toes. It’s hard!
Flex Your Muscles There isn’t a lot of room in your feet for muscles so all the major ones originate on the long bones of the leg, that is the femur, tibia or fibula. These so-called “long muscles” narrow to slender tendons near the ankle and cross over, under and around it to reach their insertion points on the metatarsals and phalanges. The “short muscles” that originate on the foot are only used for fine-tuning when you stand and walk, distributing pressure and adjusting to uneven ground.
Math Fun Foot bones are occasionally used by forensic scientists to estimate stature when long (leg) bones are unavailable. Here’s a formula Byers et al. (1989) developed for the third metatarsal from a sample of contemporary American adults. Try it on our adult sloth. Measure the length of the bone down its central axis.
Stature (inches) = 13.6 X length (in inches) + 28.3 ± 5.3
Compare your answer to the stature estimates we got using other bones. (radius lesson, ulna lesson, clavicle lesson) [The metartarsal is 61 mm. long or about 2.4 inches. That translates to a height estimate of about 61 inches, or just over 5 feet tall. We said before that sloth metatarsals were small, the opposite us. Here’s further evidence. This metatarsal is short, even for a human. The bone is weight-bearing, so its size should be related to the animal’s height and weight, but clearly the human formula is inappropriate.]
Listen like a sloth–with your feet Big feet are useful for more than just walking. Some animals actually hear with them. All animals have vibration sensors in their legs and feet signaling the brain and muscles about how firm the ground is and how hard they are stepping. Some animals put those sensors to work listening for the vibrations of prey (e.g. cats) and/or partners. Sloths may have communicated through the ground by stomping out territorial warnings or love-calls, listening for responses with their feet, the way elephants do with their feet and trunks! (Blanco and Rinderknecht, 2008) Low frequencies travel farther through the ground and are unaffected by foliage and other obstacles on the surface. How sensitive are your fingers to vibrations when someone drums a table? Close your eyes and try it. How sensitive are your feet? Have your students stand up and turn their backs to you. Now rock on your feet and strike the ground with your heels. Can they feel the vibrations? Move around the room. Can they detect the direction? The problem is figuring out how far away a signal source is, and in what direction it lies. Your ears do that with sound by sensing the small delay between when the sound arrives in one ear versus the other and the difference in loudness, but human legs aren’t far enough apart, nor are our vibration sensors sensitive enough to distinguish the differences in ground vibrations. Sloths, with widely spaced legs and the right sensors, might have been better equipped.
Walk like Frankenstein’s Monster: University of Iowa doctors are researching the problems athletes often suffer after a serious knee injury when the connections to the vibration sensors we all have in our lower legs are cut. (Madey et al., 1997) The sensors help you place your feet down gently, automatically adjusting to the ground. Without them you might walk like Frankenstein’s monster–putting your feet down too hard and slightly off balance. Try walking like Frankenstein. Pretend you don’t have your vibration sensors connected!
Stand up for sesamoids Precise data on Megalonyx’s sesamoids are scarce but data on the comparably-sized Harlan’s sloth (Paramylodon harlani) are readily available because of the large number retrieved from Rancho La Brea. They average about 46-48 mm long, 32 mm wide and 20 mm deep for adults. (Raymond and Prothero, 2010) Hold up a ruler next to the metatarsophalangeal joint to get an idea just how large the sesamoids are. Cut one out of paper, or better yet use some clay to construct models. Notice how much they change the shape of the ball of the foot.
Things to think about
Megalonyx would have been an ideal candidate for seismic communication. They had a large mass capable of producing low seismic frequencies vocally or mechanically (e.g. foot stomping), they were well coupled (connected) to the ground for optimal reception, and lived in a habitat (i.e. forests) where such communication has advantages. And we know now, because of the Tarkio Valley discovery, they were social, or at least interacted with juveniles for an extended time. Could they have listened for predators or potential mates through their big feet? Were families staying in touch that way when out of sight of each other?
Most ground sloths, Megalonyx excepted, walked on the outside of the their feet with the soles of their feet turned inward. Scientists call that pedolateral walking (see astragalus lesson). Megalonyx was unusual among ground sloths in walking like us, plantigrade, on the soles of their feet. Evidence comes from everywhere in their feet including their toes. These toe bones are much higher than they are wide, and thus stronger vertically to support the weight of the animal. Pedolateral toes are turned 90° and need to be relatively wider. Megalonyx’s toes are symmetrical around the middle digit, and the articulating surfaces are approximately equal on each side and oriented vertically. Compare the metatarsals of Megalonyx to those of the pedolateral Harlan’s sloth (left). Note how much more robust the weight-bearing digits #4-5 are in comparison to Megalonyx; compare the long articulation of the 3rd metatarsal (c) with #4 (d) versus the small articulation with metatarsal #2(b). Compare the claws. There’s no symmetry here because all the weight has been shifted outside (laterally).
The scientists who study other ground sloths point to the projection on the 5th metatarsal as further evidence of their pedolateral stance and suggest the large lateral extension is an adaptation to increases the area of support. Megalonyx doesn’t walk that way, so why does the 5th metatarsal have a similar big (weird) knob? (arrow) The knob is the base for attaching the peroneus brevis muscle. If the knob is big then the muscle had to be huge! Firmly anchored on the lateral side of the fibula (outer lower leg bone), it everts the foot, that is it bends it out (laterally)–try walking on your inner ankle bones, that’s eversion. If we believe bones grow large like this in response to the forces and stresses on them, we need to figure out what’s pushing on the 5th metatarsal so hard that Megalonyx needs to pull back on it with so much force to keep its feet down flat!
Future research
Scientists have suggested that the general hearing range of fossil animals can be determined by measuring the curvature of the cochlea, the sound-sensing organ of the inner ear, using a CT scan. (Blanco and Rinderknecht, 2008) Vertebrates generally vocalize in the same range as their hearing so estimating Megalonyx’s hearing range may provide clues about their vocal frequencies as well. (Walsh et al., 2009)
Studying sesamoids and the degree to which their growth is under genetic control versus epigenetic (i.e. environmental) factors may help scientists understand the causes of some human diseases like osteoarthritis, where tissue mineralization goes awry. Pritchett (1984) found people with an extra knee sesamoid called the fabella were significantly more likely to suffer from osteoarthritis, a degenerative joint disease. Could the growth of some sesamoids provide doctors with an advanced warning about a predisposition to diseases later in life and allow early treatment? (Sarin et al., 1999)
Conclusion
The bones of Megalonyx’s middle toe worked as a unit, playing the same role the big toe does in humans with regards to strength, balance and locomotion. This toe evolved for toeing-off, and that in itself is a remarkable insight! Far from being a lumbering, flat-footed lunk, this sloth could “tip-toe.” It’s amazing that two such small and seemingly insignificant bones can tell us so much about the shape of the animal’s foot and how it moved. Unfortunately however, by themselves these bones can’t tell us whether or not Megalonyx walked upright. Maybe the answer will be in solving another mystery our examination uncovered–what’s going on with the weird L-shaped 5th metatarsal? We may find the answer by looking a little higher, at another strangely shaped sloth bone, the femur.
TO LEARN MORE ABOUT OR TO BORROW THE UNIVERSITY OF IOWA MUSEUM OF NATURAL HISTORY GEO-2-GO DISCOVERY TRUNKS CALL OR CONTACT THE MUSEUM.
References
Aiello, L. and Dean, C. 1990. Introduction to Human Evolutionary Anatomy. Academic Press Limited. San Diego, CA
Bizarro, A. H. 1921. On sesamoid and supernumerary bones of the limb. Joiurnal of Anatomy 55: 256-268.
Blanco, E. and Rinderknecht, A. 2008. Estimation of hearing capabilities of Pleistocene Ground Sloths (Mammalia, Xenarthra) from middle-ear anatomy. Journal of Vertebrate Paleontology 28: 274-276.
Byers, S., Akoshima, K. and Curran, B. 1989. Determination of adult stature from metatarsal length. American Journal of Physical Anthropology 79: 275-279
David, R. D., Delagoutte, J. P. and Renard, M M. 1989. Anatomical study of the sesamoid bones of the first metatarsal. Journal of the American Podiatric Medical Association 79: 536-544.
Educational Technology Clearinghouse, Florida Center for Instructional Technology, College of Education, University of Southern Florida http://etc.usf.edu/clipart/
Finch, J. 2011. The ancient origins of prosthetic medicine. The Lancet 377: 548-549.
Hildebrand, M. 1985. Walking and running. In Functional Vertebrate Morphology. M. Hildebrand, D. M. Bramble, K. F. Liem and D. B. Wake (eds.) Harvard University Press, Cambridge, MA.
Inman, V. T. 1966. Human locomotion. Canadian Medical Association Journal 94: 1047-1054.
Jensen, J. A. 1988. A fourth new sauropod dinosaur from the Upper Jurassic of the Colorado Plateau and sauropod bipedalism. The Great Basin Naturalist 48: 121-145.
Leidy, J. 1860. Remarks on the structure of the feet of Megalonyx. Transactions of the American Philosophical Society 11: 107-108.
Madey, S. M., Cole, K. J. and Brand, R.A. 1997. Sensory innervation of the cat knee articular capsule and cruciate ligament visualized using anterogradely transported wheat germ agglutionin—horseradish peroxidase. Journal of Anatomy 190: 289-297.
McDonald, H. G. 1977. Description of the osteology of the extinct gravigrade edentate Megalonyx with observations on its ontogeny, phylogeny and functional anatomy. Master’s Thesis. University of Florida.
McDonald, H. G. 1995. Gravigrade Xenarthrans from the early Pleistocene Leisey Shell Pit 1A, Hillsborough County, Florida. Bulletin of the Florida Museum of Natural History 37 Pt. II (II): 345-373.
McDonald, H. G. 2003. Xenarthran skeletal anatomy: primitive or derived? Senckenbergiana Biologica 83: 5-17
Pritchett, J. W. 1984. The incidence of fabellae in osteoarthritis of the knee. Journal of Bone Joint Surgery 66-A: 1379-1380.
Raymond, K. R. and Prothero, D. R. 2010. Comparative variability of intermembranous and endochondral bones in Pleistocene mammals. Palaeontologia Electronica 13 (1); 4A: 14 p.
Sarin, V. K., Erickson, G. M., Giori, N. J., Bergman, A. G. and Carter, D. R. 1999. Coincident development of sesamoid bones and clues to their evolution. The Anatomical Record 257: 174-180.
Stock, C. 1917. Structure of the pes in Mylodon harlani. University of California, Bulletin of the Department of Geology 10: 267-285.
Walsh, S. A., Barrett, P. M., Milner, A. C., Manley, G. and Witmer, L.M. 2009. Inner ear anatomy is a proxy for deducing auditory capability and behaviour in reptiles and birds.; Proceedings of the Royal Society B 276: 1355-1360.